Making nerve models easier to test.
Hydrogel “nerve-on-a-chip” constructs mounted in my novel electrophysiology rig to test their response to electrical stimulus.
I spent four out of the five years I was at Tulane University, getting my Bachelor’s and Master’s degrees in Biomedical Engineering, in Dr. Michael Moore’s lab, the Neural MicroEngineering Laboratory. The lab focused on making better models of neural tissue for drug and disease research, and was a great fit for me considering my general (at the time) interest in the nervous system.
My first year in the lab, I picked up the core techniques the lab used to make these “hydrogel neurite constructs”: roughly 6mm by 4mm by 1mm tall neural culture models, with a gel in which neural tissue can grow in the middle, and one in which they can’t on the outside, patterned with light using a photolithography rig.
The part that interested me the most about the development of these models was seeing the neural tissue functioning: electrophysiology - stimulating the neurons with electricity and reading their biological electrical response. The only way the lab team had been able to achieve this was essentially by cutting the construct out of the cell culture insert, moving it to a complicated electrophysiology setup, and taking a long time to precisely poke it with probes.
A hydrogel neurite construct cut out, placed, and with probes positioned for field potential recording.
Image from Renee Huval’s work getting the first electrophysiology results from the constructs.
Electrophysiology is the best way to see how well neural tissue functions. If these neural models were ever going to help with disease research or drug discovery, doing electrophysiology on them this way was not going to cut it.
The first approach Dr. Moore encouraged me to attempt in order to improve the electrophysiology process was by making sections of the gels the neural tissue could grow in more conductive, by introducing an additional conductive polymer to form an interpenetrated network hybrid conductive hydrogel.
Unfortunately, the neural tissue simply didn’t want to grow into it. I did conduct impedance spectroscopy testing to precisely see the difference in the gels’ conductivity (and any signal distortion it might introduce) by adding the additional conductive polymer, and while there was some improvement to be had, overall it was clear the effort to prepare the portions of hybrid gel was not worth the conductivity gains.
A cylindrical hybrid conductive hydrogel sample loaded into a testing rig I designed for impedance spectroscopy testing.
(To the electrically inclined in my audience: the plate overlap only introduced 2pF of capacitance and had no apparent effect on the resulting data.)
Since the hybrid gel approach didn’t work, it was clear that improved electrodes was going to be the way forward to making electrophysiology scalable for the hydrogel constructs. At the time, commercial planar electrode arrays were becoming available, though they were expensive, and moreover, were incompatible with the way we kept the neurite constructs fed, through a permeable membrane below them.
One of the cell culture inserts the lab used for making hydrogel neurite constructs, with a permeable membrane on the bottom. Six of these would fit into a plate, with wells underneath each for holding culture media.
And so it was that I needed to design improved electrodes, and an accompanying interfacing rig, that:
Was completely compatible with the permeable cell culture inserts.
Was faster to use than manually probing the constructs.
Could actually stimulate and record the biological response of the neural tissue in the constructs (AKA “works”).
To make this happen, I had to design “A Platform for Rapid Electrophysological Analysis of Hydrogel Neurite Constructs”, as I called it in the title of my Master’s thesis.
The first major part of this platform were the electrodes themselves. With assistance from another department, I was able to experiment with depositing metal directly onto the surface of the inserts using Physical Vapor Deposition - which sort of works how it sounds - shooting a vapor of metal in a vacuum chamber at a target.
Once I was able to confirm the pores were still there after deposition, and thus the membranes were still permeable, I had to figure out how to make the electrode pattern in the inserts.
After a lot of prototype iterations, I landed upon a design that worked: 3D printed components that would snap directly into the cell culture inserts, holding in place a precise, CNC machined mask pattern in copper. The last to-do was an apparatus to hold several inserts in place at once, with their snap-in masks, for metal deposition.

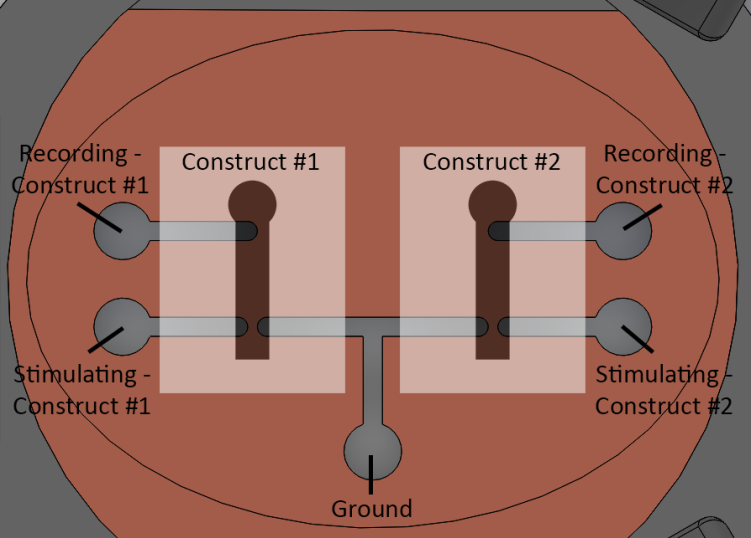
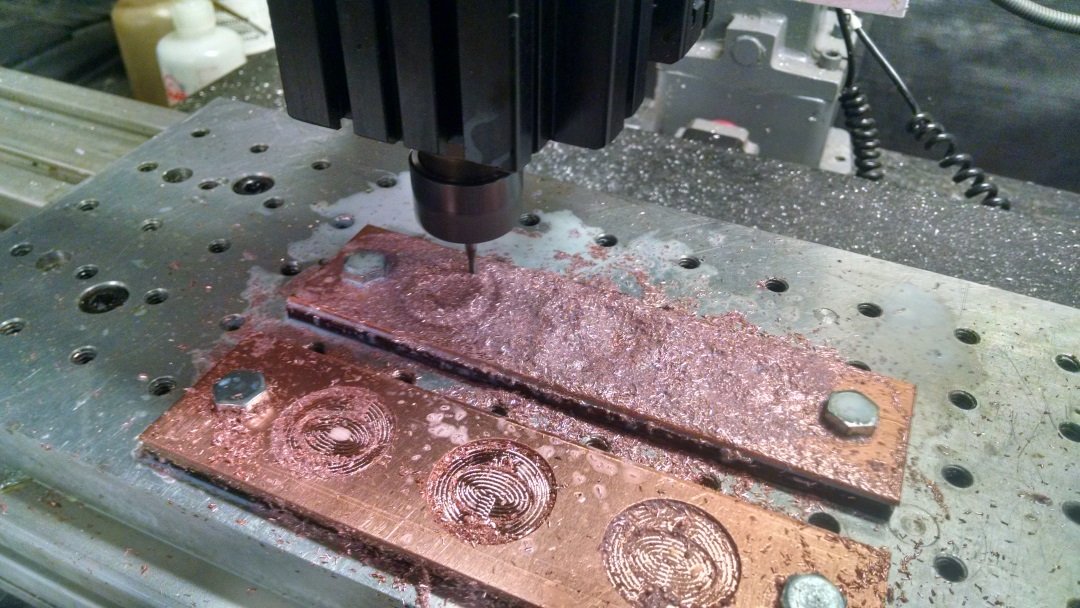
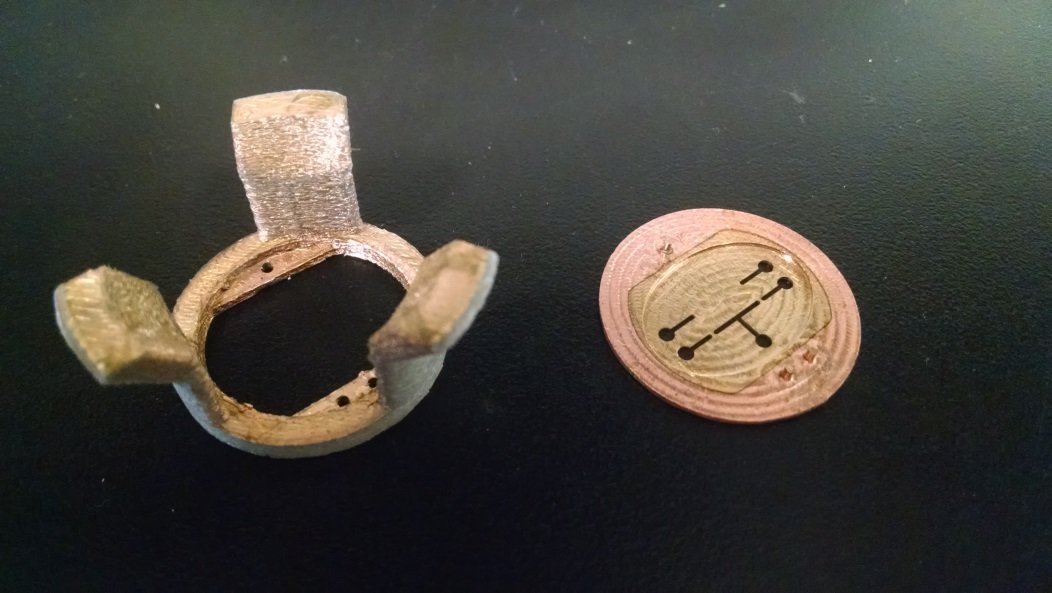
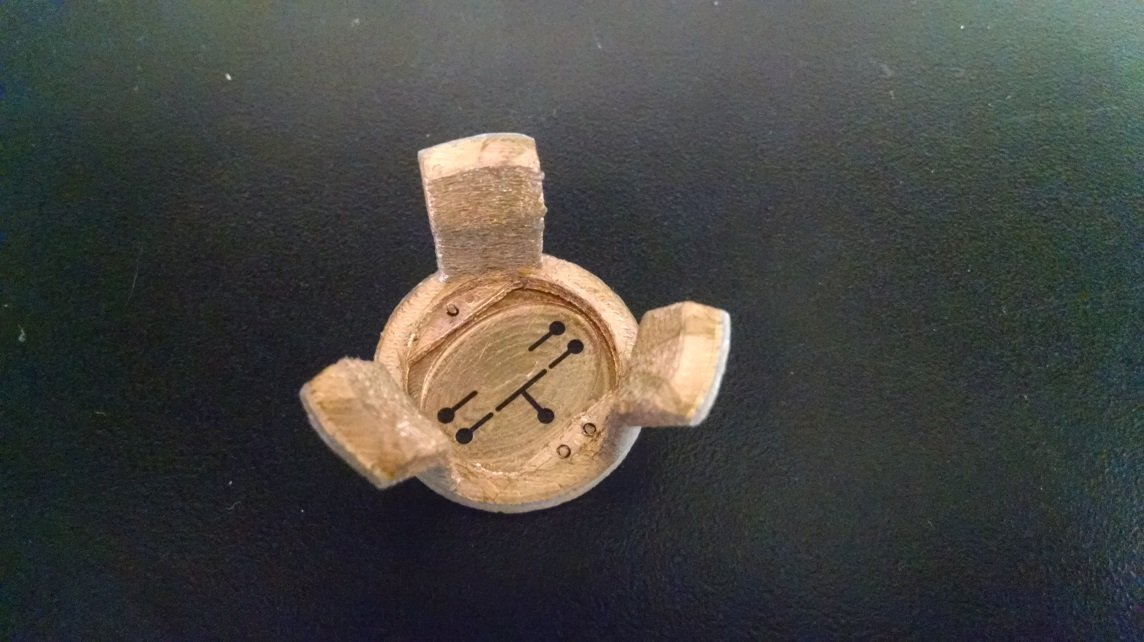
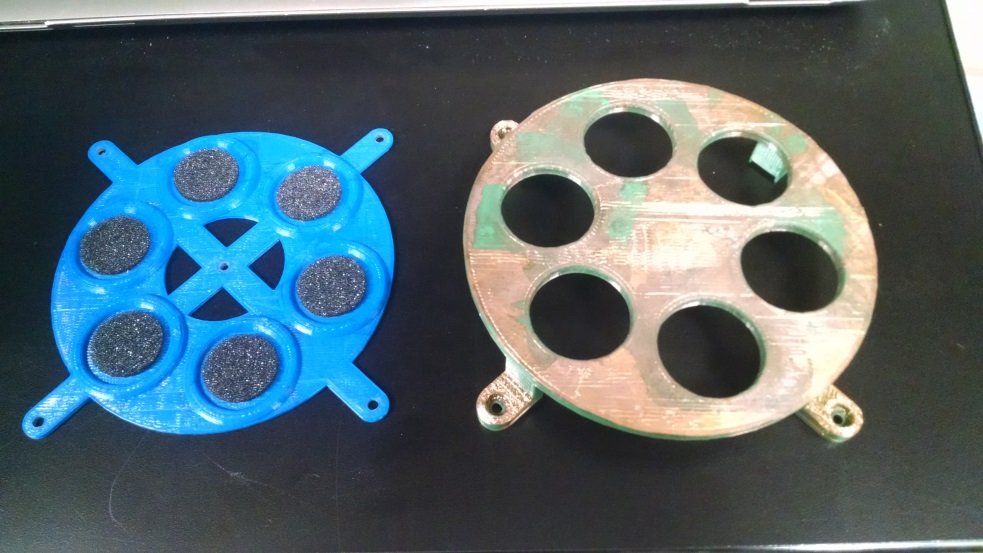
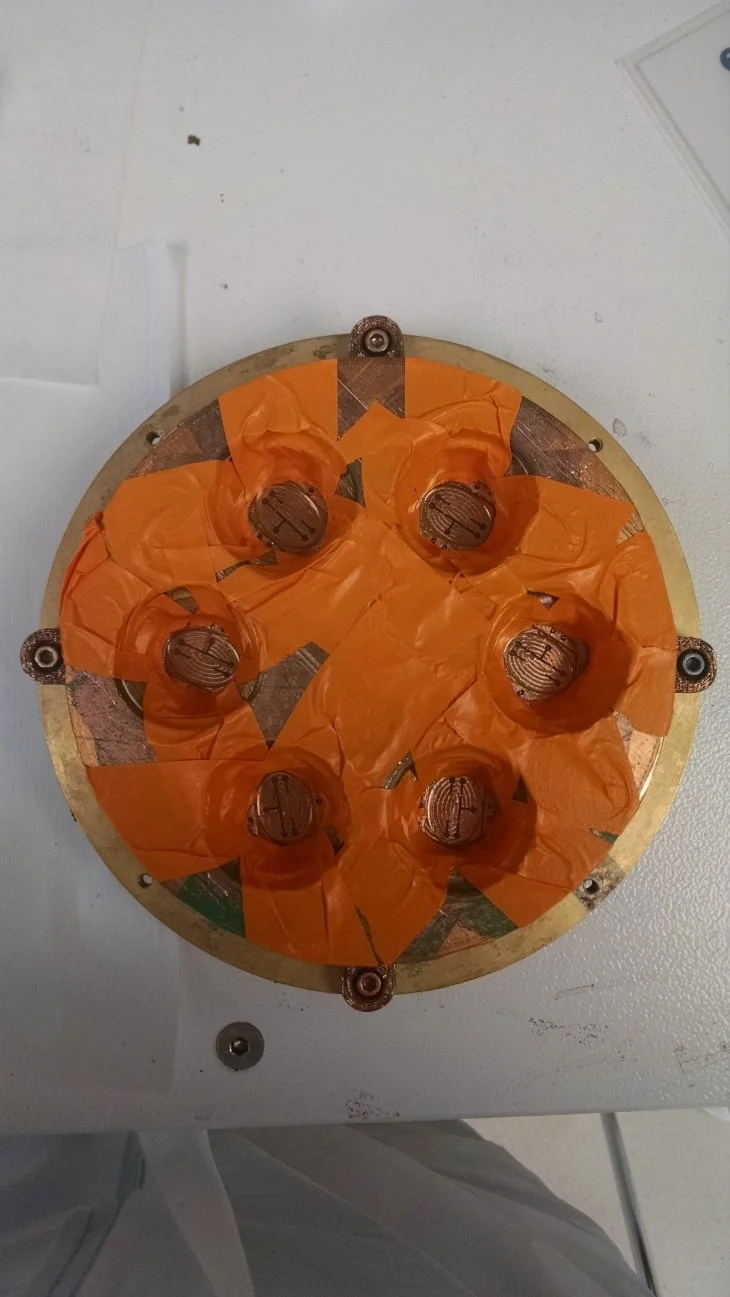
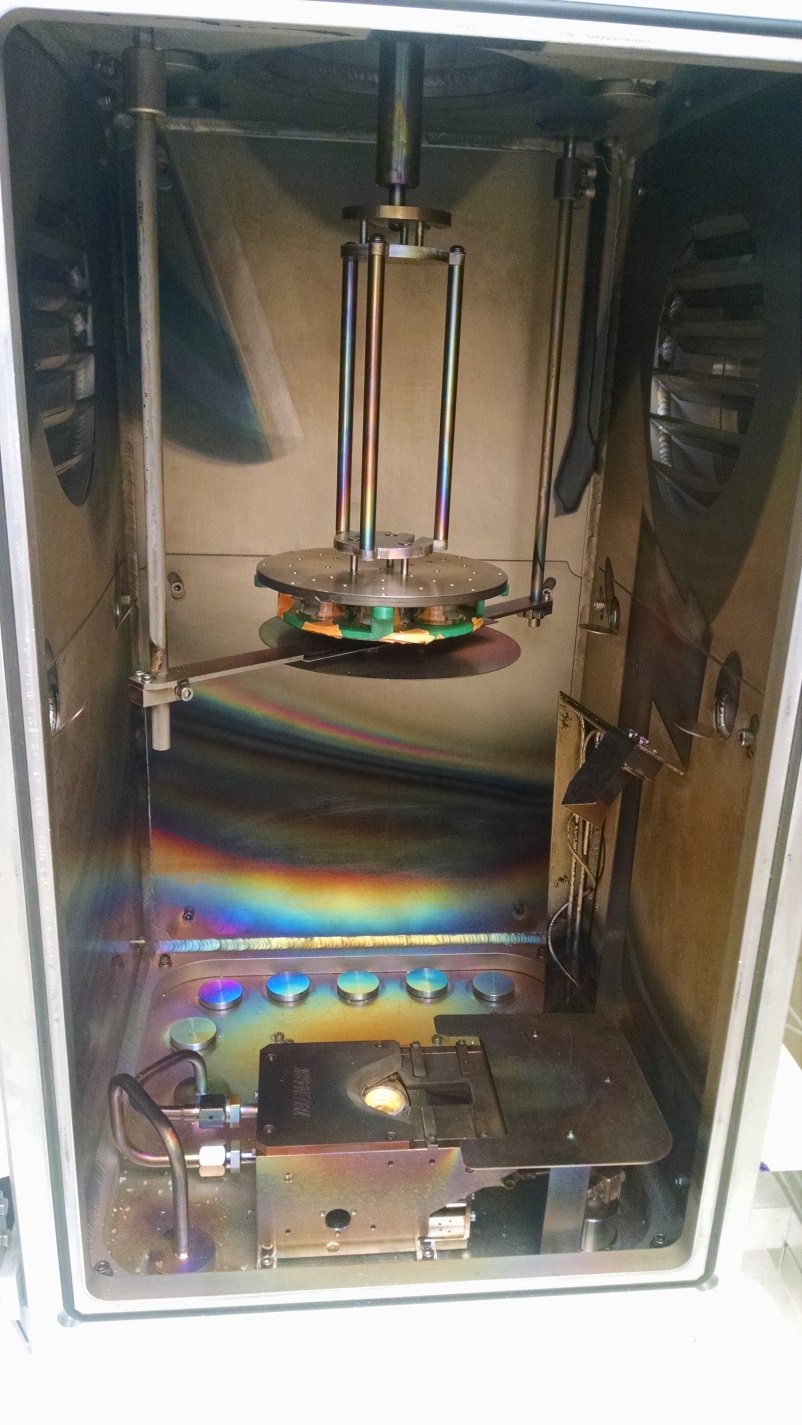
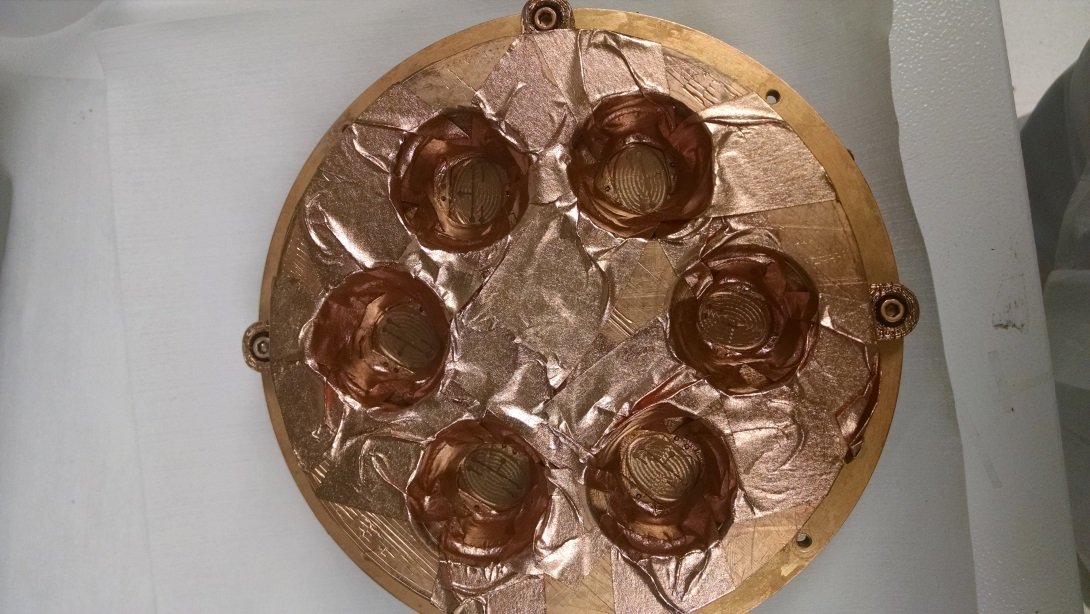
Cell culture inserts with successfully deposited electrode pattern!
With the electrodes patterned, the other piece of the puzzle was electrically interfacing with them. Since the snap-in masks controlled the orientation of the electrode pattern based on three holes around the walls of the inserts, I could design a rig that would also use one of those holes to orient the inserts the correct way, and bring down pins directly onto the contact pads on the inserts.

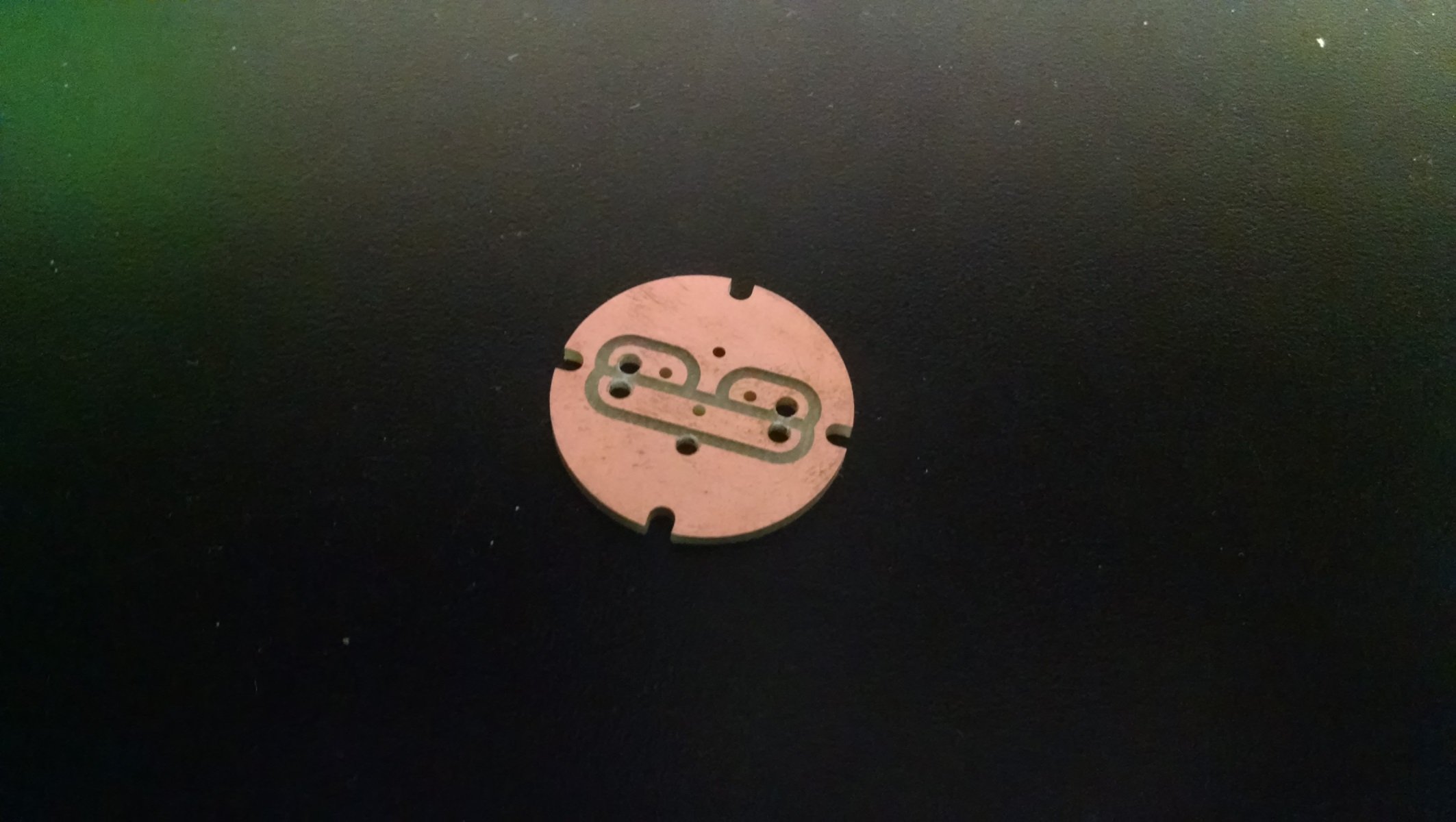
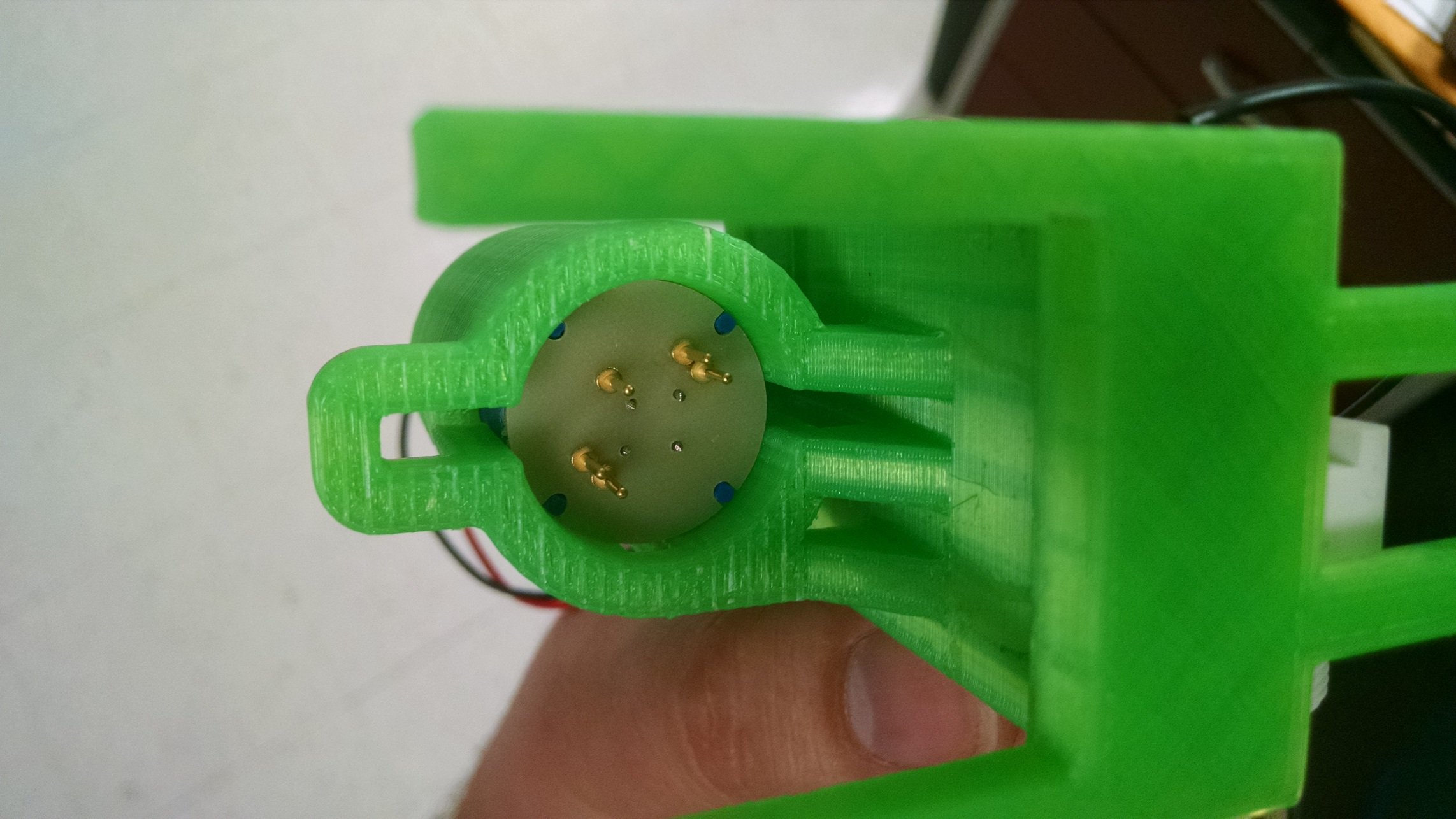
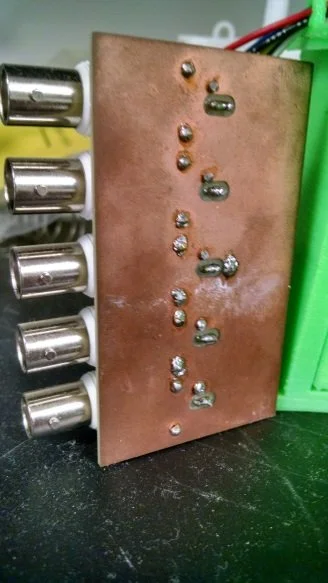
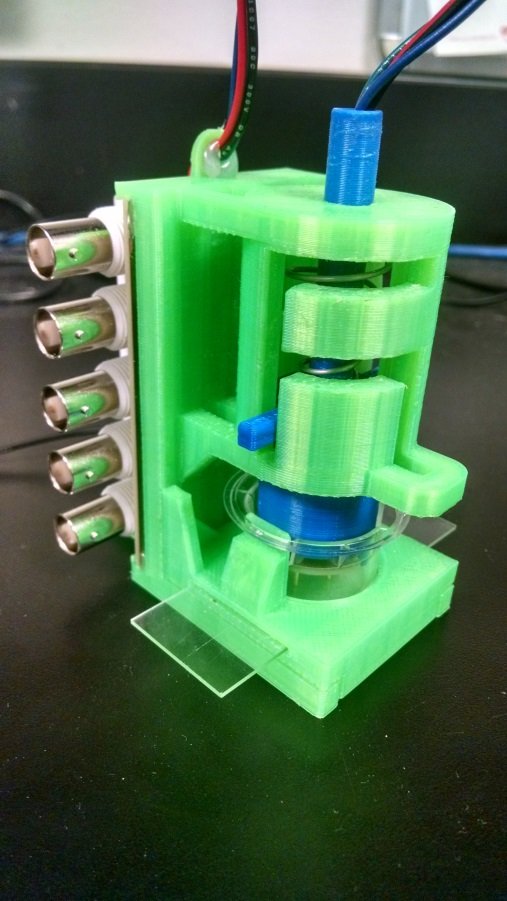
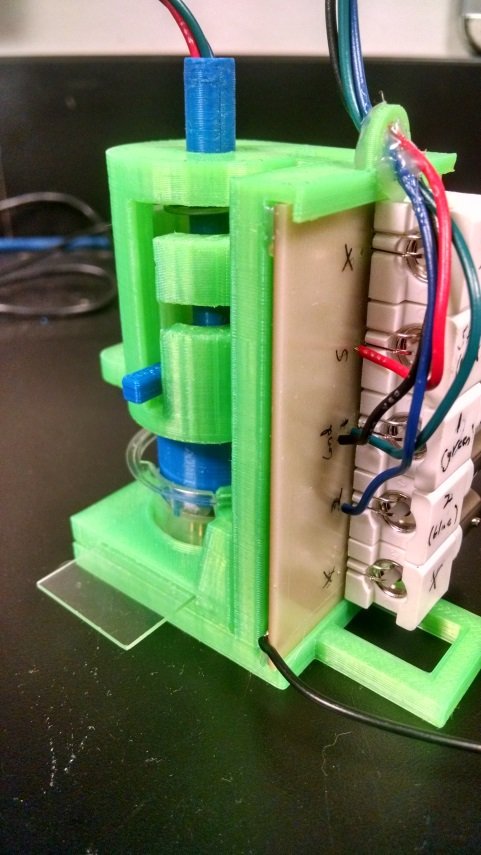
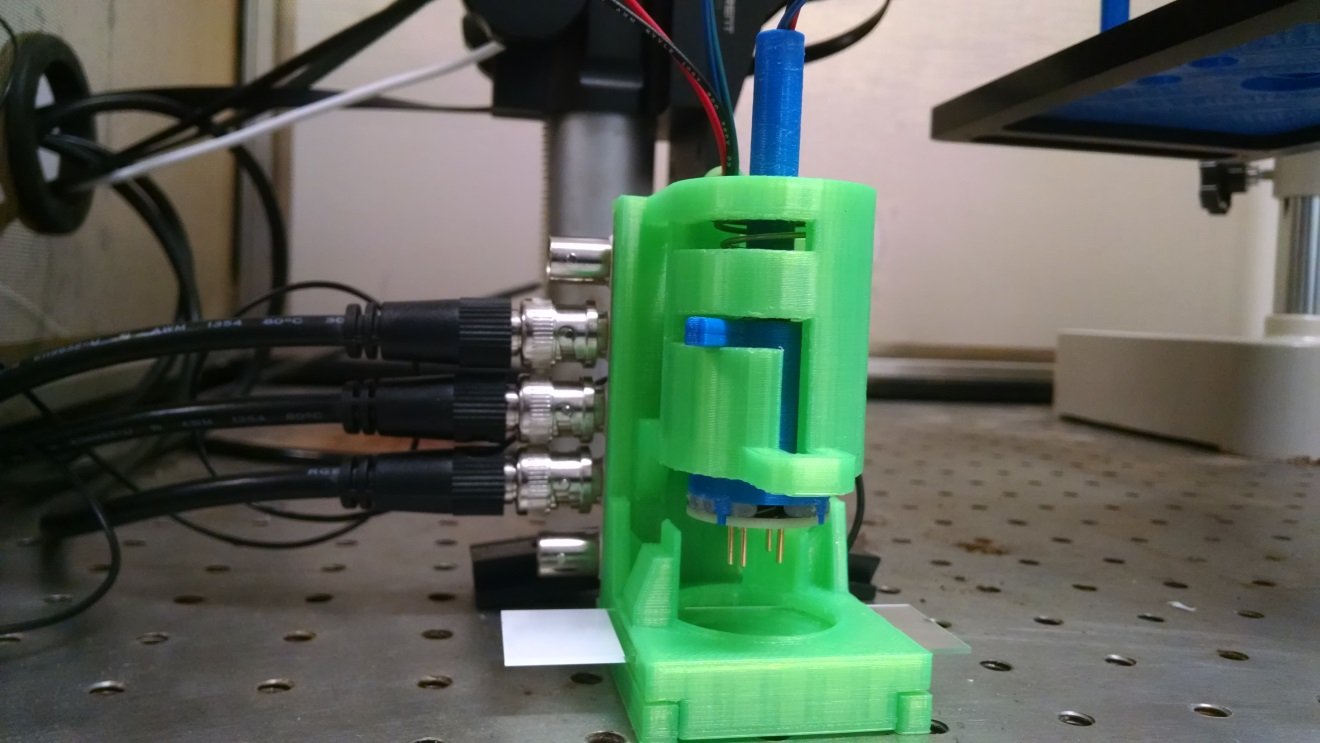
Detail view of the headline image: neurite constructs ready for electrophysiology in the rig.
Well, in short, it worked! The electrode pattern I was able to make on the cell culture inserts, when interfaced with using my rig, was able to stimulate and record biological responses from neurite constructs. Here’s what that looked like:
The dips in the blue line are the responses to the stimulus.
And here are pictures of the neural growth in the constructs from which I was able to confirm biological responses:
My work on improving electrophysiology throughput for hydrogel neurite constructs is currently being built upon in Dr. Moore’s startup for commercializing “nerve-on-a-chip” models: Axosim.
If you’re interested in learning more about my Master’s thesis research, you can read the whole thesis document here, or look into the related patent filings here.
Official record in the Tulane University Digital Library here.